Technology |
Improved catalyst turns harmful greenhouse gases into cleaner fuels, chemical feedstocks
A chemical reaction can convert two polluting greenhouse gases into valuable building blocks for cleaner fuels and feedstocks, but the high temperature required for the reaction also deactivates the catalyst. A team led by the Department of Energy’s Oak Ridge National Laboratory has found a way to thwart deactivation. The strategy may apply broadly to other catalysts.
With an improved catalyst, ORNL chemists converted two greenhouse gases, methane (CH4) and carbon dioxide (CO2), to syngas, a valuable mix of hydrogen (H2) and carbon monoxide (CO). Photo: Philip Gray/ORNL, U.S. Dept. of Energy
The team improved a reaction called dry reforming of methane that converts methane and carbon dioxide into syngas, a valued mixture of hydrogen and carbon monoxide used by oil and chemical companies worldwide.
“Syngas is important because it's a platform for the production of a lot of chemicals of mass consumption,” said ORNL’s Felipe Polo-Garzon, who, with ORNL’s Junyan Zhang, led the study.
Improving the catalyst that speeds syngas production could have enormous impact on global energy security, cleaner fuels and chemical feedstocks. In countries lacking oil reserves, syngas derived from coal or natural gas is critical for making diesel and gasoline fuels.
Moreover, syngas components can be used to make other commodity chemicals. Hydrogen, for example, can be used as a clean fuel or as a feedstock for ammonia to create fertilizer.
Methanol, an alcohol that can be made from syngas, is a source of ingredients for producing plastics, synthetic fabrics and pharmaceuticals. Methanol is also a good carrier of hydrogen, which is hard to pressurize and dangerous to transport.
As the simplest alcohol, methanol contains the highest ratio of hydrogen to carbon; it can be safely transported and converted to hydrogen at the destination.
“This [dry reforming of methane] reaction sounds attractive because you are converting two greenhouse gases into a valuable mixture,” Polo-Garzon said. “However, the issue for decades has been that the catalysts required to carry out this reaction deactivate quickly under reaction conditions, making this reaction nonviable on an industrial scale.”
To attain significant conversion of reactants, the reaction must be conducted at temperatures greater than 650 degrees Celsius, or 1,200 degrees Fahrenheit.
“At this high temperature, the catalysts undergo two deactivation processes,” Polo-Garzon said. “One is sintering, in which you lose surface sites that undertake the reaction. The other is the formation of coke — basically solid carbon that blocks the catalyst from contacting the reactants.”
Catalysts work by providing a large surface area for reactions. Metal atoms such as nickel have electronic properties that allow them to temporarily bind reactants, making chemical bonds easier to break and create. Sintering causes nickel particles to clump, reducing the surface area available for chemical reactions.
Likewise, coking chokes a catalyst. “During the reaction on the catalyst surface, methane will lose its hydrogen atoms one by one until only its one carbon atom is left,” Zhang said.
“If no oxygen bonds to it, leftover carbon will aggregate on the catalyst’s nickel surface, covering its active face. This coking deposition causes deactivation. It is extremely common in thermal catalysis for hydrocarbon conversion.”
Today, most commercial syngas is made by steam reforming of methane, a process that requires large amounts of water and heat and that also produces carbon dioxide. By contrast, dry reforming of methane requires no water and actually consumes carbon dioxide and methane.
By tuning interactions between the metal active sites and the support during catalyst synthesis, the scientists suppressed coke formation and metal sintering. The new catalyst provides outstanding performance for dry reforming of methane with extremely slow deactivation.
The novel catalyst consists of a crystalline material called a zeolite that contains silicon, aluminum, oxygen and nickel. The zeolite’s supportive framework stabilizes the metal active sites.
“Zeolite is like sand in composition,” Zhang said. “But unlike sand, it has a sponge-like structure filled with tiny pores, each around 0.6 nanometers in diameter. If you could completely open a zeolite to expose the surface area, 1 gram of sample would contain an area around 500 square meters, which is a tremendous amount of exposed surface.”
To synthesize the zeolite catalyst, the researchers remove some atoms of aluminum and replace them with nickel. “We're effectively creating a strong bond between the nickel and the zeolite host,” Polo-Garzon said. “This strong bond makes our catalyst resistant to degradation at high temperatures.”
Zhang performed infrared spectroscopy, revealing that nickel was typically isolated and bound by two silicon atoms in the zeolite framework.
The high-performance catalyst was synthesized at ORNL’s Center for Nanophase Materials Sciences. Zili Wu, leader of ORNL’s Surface Chemistry and Catalysis group, served as a strategy advisor for the project.
Zhang performed infrared spectroscopy, revealing that nickel was typically isolated and bound by two silicon atoms in the zeolite framework.
At DOE’s Brookhaven National Laboratory and SLAC National Accelerator Laboratory, ORNL’s Yuanyuan Li led X-ray absorption spectroscopy studies detailing the electronic and bonding structures of nickel in the catalyst.
At ORNL, Polo-Garzon and Zhang used a technique called steady-state isotopic transient kinetic analysis to measure catalyst efficiency — the number of times a single active site converts a reactant into a product. (Oak Ridge National Laboratory)
YOU MAY ALSO LIKE



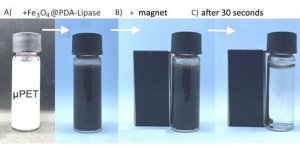

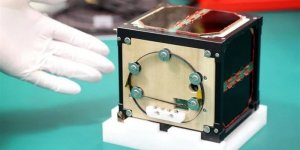

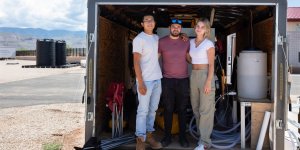

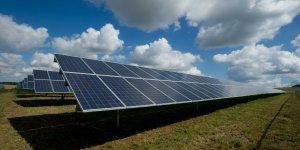


